Aluminium and heat treatment
Aluminium as construction material
Aluminium was discovered in the early 19th century, but it took till 1886 to develop a commercial extraction proces. Before that aluminium was a very expensive metal. It's light weight, made it a potential metal to replace steel in bike frames. The softness and lack of strength and stiffness, made that difficult for early designers: most frames failed within a short time. The lack of a good construction method was one of the problems.
The St.Louis Refrigerator and Wooden Gutter Co., St.Louis, Missouri, made the Lu-Mi-Num bicycle in the early nineties of the 19th century; the frame was cast in one hollow piece. The front fork was cast solid.
The rights to the manufacture of the Lu-Mi-Num bicycle were purchased by:
M.M. Cycles, Sans Souderes en Lu-Mi-Num ( Paris).
It was sold in England by the Lu-Mi-Num Mfg. Co., Ltd. of London.
Lu-Mi-Num's 1895 ladies and gents models weighed 26 lbs. and were sold for $125.
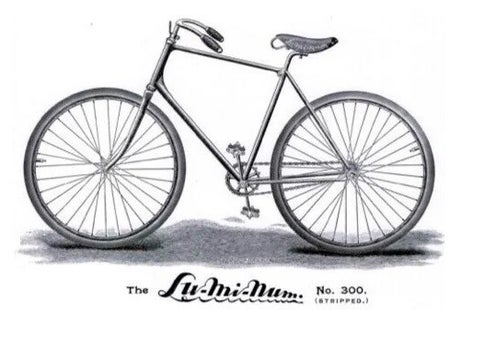
FIG.1 Lu-Mi-Num cast bike frame.
In England the first manufacturer was Humber: the Brazeless in 1895. This was an attempt to use a cotterpin construction method. Some people have used the bike for several years, but no known surviving frame exists. The Lu-Mi-Num jointless cycle (1894) used a casting process. We can see advertisments from the USA and from Paris (licencesed), see FIG.1 and 2 Also in Paris: the firm Rupalley FIG.3 , they manufactered welded aluminium bikes in the 1895-1900 era; the advertising of mr. M.Corre riding around France is well known.
In 1924 the German firm Diamant made lugged/pressed/rivetted aluminum bikes for their racing teams (Reichspat.437305); as they used thick-walled tubes, these frames were not much lighter than their steel racers (over 2kg), but lasted as long.
The rise of the aviation industry increased the demand for aluminium. Better and cheaper electrical melting processes encouraged the development of new alloys. Between the wars a lot of sciencists worked to make aluminium succesful. Many alloys with fancy names were produced in those days, like: Silumin (alu-silicium alloy), Dural(uminium) an alu- copper alloy, Hiduminium (an alu-nickle alloy) and Electron (an alu-magnesium alloy). Some alloys, like Dural and Hidunium could not be welded and were mechanically bonded.
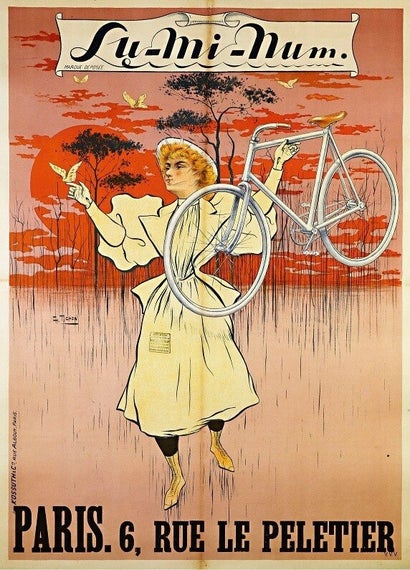
FIG.2 As light as a feather....
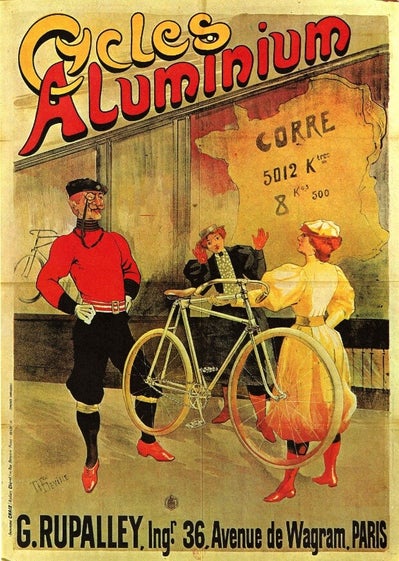
FIG.3 Or is it a strong Paris woman?
In France there have been a lot of companies in the thirties using clamps, bolts, and rivets. Pelage (1933) used the clamp / rivet design by M.G. Py (see FIG4.b)
The company Caminade (see FIG.4a) built bikes with octagonal Dural tubes, bolted and clamped with octoganal lugs (1935). Dural is a strong but not weldable alloy. Meca Dural, Duralavia, Gnome-Rhone , Aviac were other French manufacturers using similar methods during the forties.
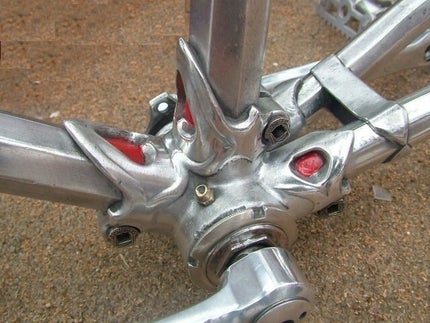
FIG.4a Caminade construction detail (photo: Classic Rendezvous)
In 1934 the French builder Pierre Colin made frames as light as 1,1 kg; but these were not durable.
An unemployed aviation engineer, started building aluminium bikes too: Nicola Barra. He welded his frames of an aluminium/ magnesium alloy with the flame. A superb craftsman who built aluminium bikes till the early fifties.
In 1950 the London firm Fonteyn advertised with Fontalloy frames. Holdsworth also experimented with a welded frame in those days, one of the Barra look-a-likes from the late forties. Even large French bike manufacturers started offering those welded frames.
Then suddenly the market for these bikes declined; too expensive??
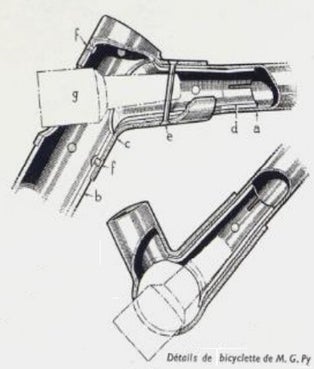
FIG. 4b Pelage construction details.

FIG. 4c Dural construction method by Aviac.
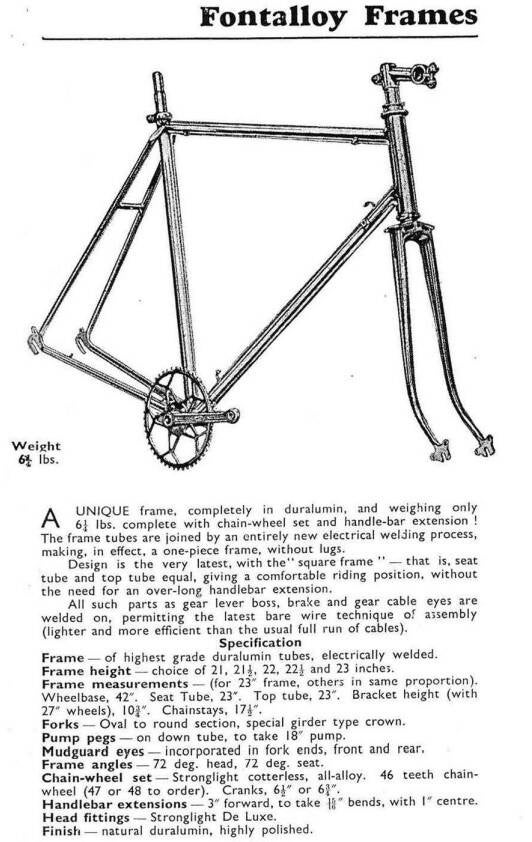
FIG.5 This frame was marketed by Fonteyn in 1950
The German Hermann Klaue designed this cast crossframe (1950). It was made of Silumin and it was succesfull; certainly after the take-over by Hercules in 1956. It was in production till 1964 in and it was the first alu bike for the commuter. In the sixties this design was marketed as "uni-sex" .
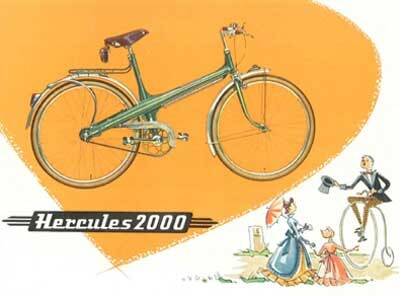
FIG.6a The German engineer H. Klauwe designed this cast aluminium bike in 1950.
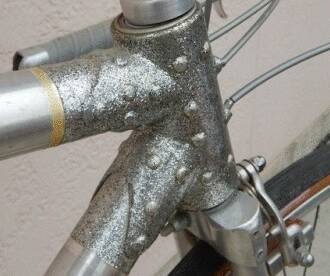
FIG.6b Hi-E rivetted lugs (photo: Classic Rendezvous).
The American Harlan Meyers made very light bikes in the early 70's (1200gr.). He named his frames Hi-E ; he made his own tubes, lugs, rims, hubs, crankset and pedals , see FIG.6c.
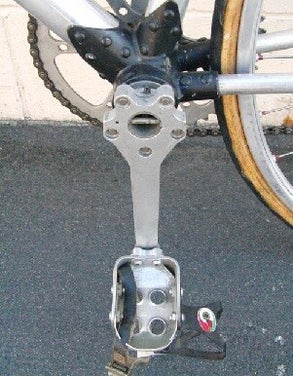
FIG.6c Hi-E bracket and crankset (photo: Classic Rendezvous).
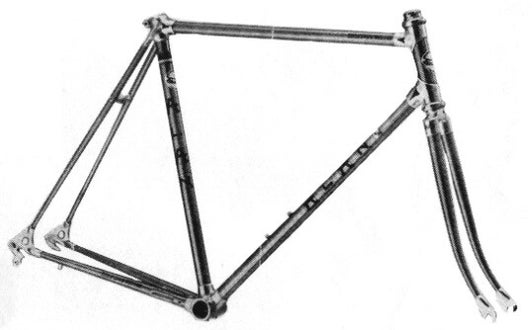
FIG. 7a Alan 1972: aluminium, glued and screwed.
The Swiss engineer Hans Müller patented a frame construction with threaded lugs and tubes in '34 . Probably it was never used.
The Italian Alan racers were made that way in 1972 : glued and screwed, not superlight . They were sold under many names......
Around 1980 the French firm Vitus also built glued frames with Vitus tube and CLB castings. The tube was slid over the ends of the casting and bonded with Araldit, a two-component epoxy adhesive commonly used in the aircraft industry. After gluing, the frame was cured in an oven. The adhesive surface is quite large, because the strength of the adhesive bond per cm² is only 10% of a brazed joint. In later years that made the step to carbon tubing easier. In 1983 we see the first variants with the same construction (like the Peogeot PY-10) but now with the three main tubes made of carbon.
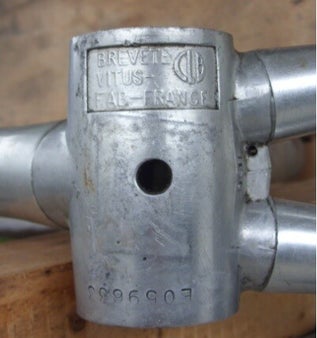
FIG.7b Cast alu bracket from CLB (Vitus)
In France, the craftsman André Sablière made beautiful welded aluminum frames in the 1980s. The German company Kettler even started mass production of robotically welded aluminum bicycles 1977. Unfortunately, the Kettler Alu-rad 2600 ran into many technical problems (no heat treatment?). The frames were recalled and withdrawn from the market.
Peugeot made a "pressed clamped" aluminum bicycle (FIG.7d) in the mid range of the market in 1987, but it silently disappeared after a year.
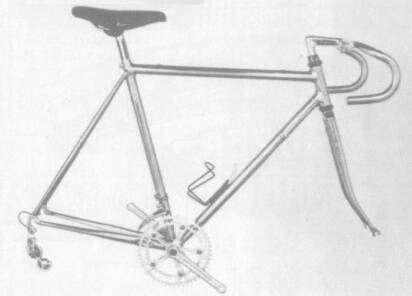
FIG.7c Heli-arc welded Zicral frame from André Sablière early eighties.
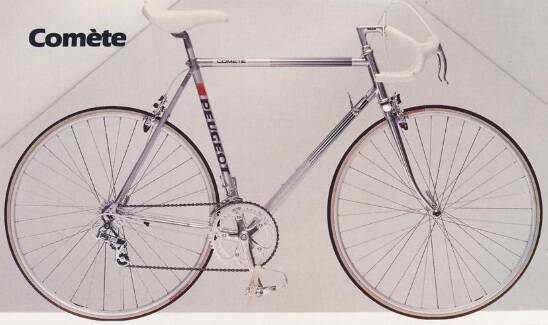
FIG.7d The Peugeot Comete was a "pressed clamped" frame (1987}.
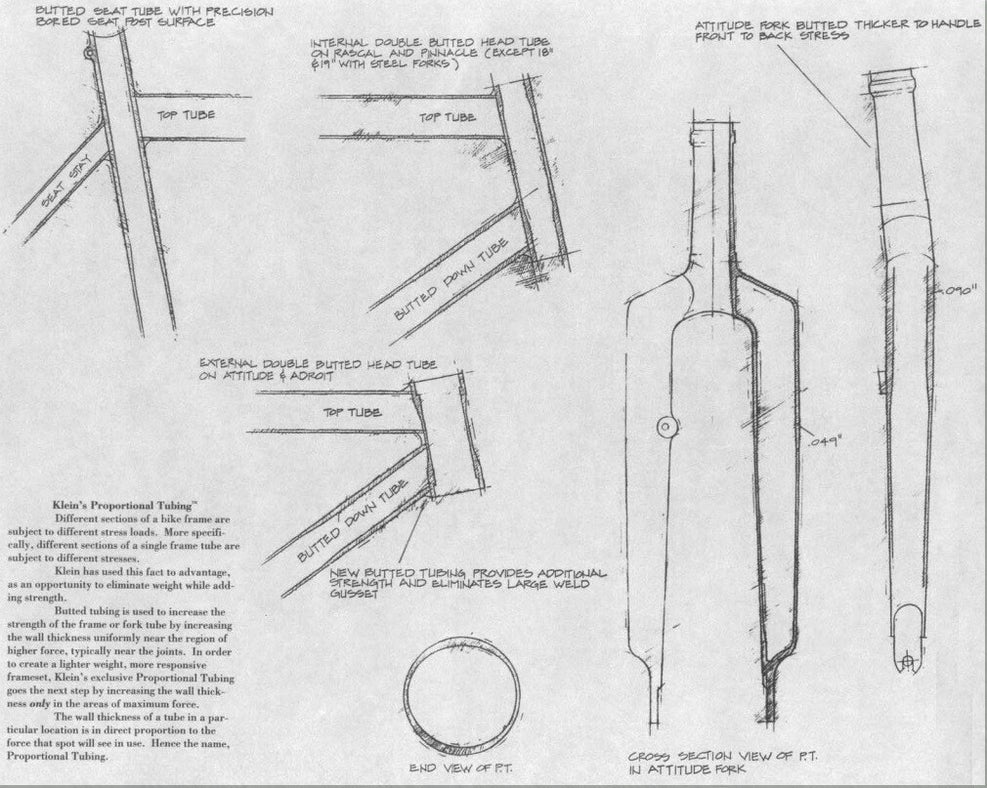
FIG.8a This is a drawing of the Klein frame construction, showing reinforcements and weldseams (1991).
Without a doubt, Gary Klein introduced the oversized tubes and TIG-welded aluminium bicycles in the USA in the 1970s. This started a revolution in the bike industry worldwide.
Cannondale began mass production of this concept in the early 1980s (lower price and quality); others followed.
When the ATB hype exploded in the late 1980s, the US started outsourcing to Asia. Europe's bicycle industry outsourced their production years later.
China and Taiwan became the world's leading manufacturers of aluminium bicycles.
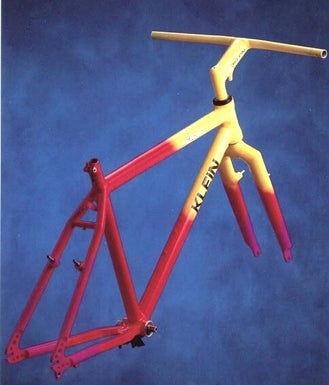
FIG.8b Klein Adroit ATB 1991
The heat treatment of aluminium
In a homogeneous molten metal mix, all molecules are in solution. While cooling, some elements start to solidify. When crystals are formed, some elements will be pushed out (precipitated). This leads to a lot of pressure and deformation inside the grain structure.The metal gets harder, and more force is needed to bend or deform it. We call this strength; when pulling, elongation before breaking will always be less. Reheating will undo the precipitation, as it will dissolve the elements again. Different temperature drops create other patterns of precipitation, so welding and brazing can influence the resulting strength.
There are various methods to improve the strength of metals, such as alloying (mixing) and heat treatment. To understand how this works, we need to look at different types of microstructures in solids. Glass is amorphous, i.e. the molecules do not have regular positions with respect to each other. Most substances, including metals, are crystalline, i.e. the atoms are arranged in fixed patterns. This can be done according to three structures: 1. Face centered cubic or fcc ( copper, alpha iron); 2.Body centered cubic or bcc ( gamma iron, molybdenum); 3. Hexagonal close packed or hcp ( includes beryllium, magnesium).
The fcc and hcp structure are maximally dense stacks: the filling level is 74%; in the bcc structure the filling level is 68%. So there are always gaps in every molecular structure, the so-called intersitial spaces; these may contain other atoms. In a stack of atoms, there may be an empty space (a vacancy) or a foreign atom (a substitution). With a general term we call these errors “dislocations”. When the temperature rises, the number of vacancies increases due to the movements of atoms. Substitutional and intersitial atoms can move through vacancies (diffusion).
Self-displacing dislocations are developed in metals under load; these deform the grid so much that they hinder their own movement. More and more energy must be supplied for further deformation; that is why the fracture energy of metals is so high. The deformation of the grid explains the reinforcement that occurs: hardness and tensile strength increase, elongation decreases. All foreign atoms affect the dislocation movements. In this way, the yield strength and tensile strength increase by alloying (mixing of metal molecules), especially if those atoms occur in groups. This is achieved, among other things, by heat treatment (the diffusion is faster that way)!
So by alloying, one can improve the material properties of metals; other methods for this are work hardening (mechanical processing, including the “drawing” of tubes), or heat treatment. Often a combination of these methods is chosen. The crystal structures of alloys are important for their properties.
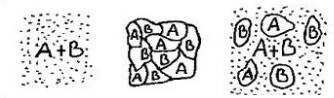
FIG.7a FIG.7b FIG.7c
If we have an alloy consisting of metals A and B, there are 3 possibilities:
1. Metal A and B dissolve into each other (FIG.7a ).
2. Metal A and B do not dissolve (FIG.7b ).
3. Metal A and B partially dissolve into each other (FIG. 7c).
Usually in alloys we are dealing with the latter structure. We take for example a mixture of metal A melting point 550 ° C and B 600 ° C. Suppose our crucible has a temperature of 700 ° C; we let it cool down slowly. In the melt, molecules A and B are evenly distributed in the liquid. First, B crystals will form. During this process, the concentration of the A molecules in the matrix (liquid) will increase, until the moment when A crystals start to form. Eventually a matrix is formed, consisting of A and B molecules, containing crystals A and B. The concentration of A and B in the matrix will not be equal to the original percentages in the mixture.
The mechanical properties will be different. If we cool very quickly e.g. in water, the alloy will be most homogeneous (a very fine crystal structure). The longer the cooling time, the larger the crystals will be.
During heat treatment the first stage is the solution treatment; we heat to a certain (high) temperature. As an example we take aluminium from the 6000 series; this is heated to 535 ° C for half an hour (during this process all kinds of alloy elements dissolve). The second stage, the precipitation treatment: we cool down quickly. The result is supersaturation and there is separation (= precipitation) of alloying elements in the crystals. These newly excreted substances will refine and distort the crystal structure. This makes the alloy harder and stronger. That will improve over time, we call this aging.
We can speed up the aging by carrying out a third stage: artificial aging.
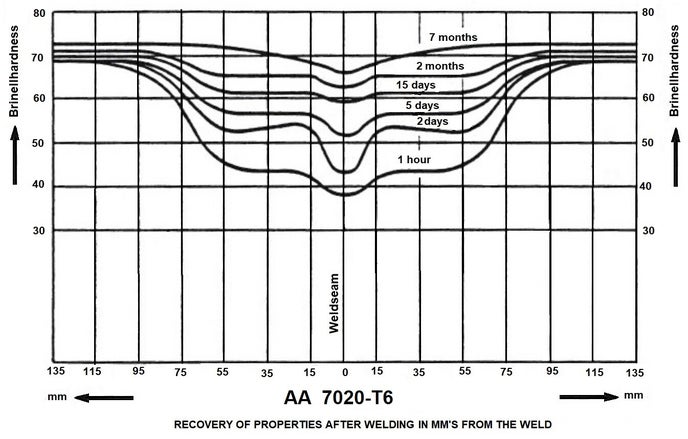
After the solution treatment, we will artificially age 6000 aluminium at 180 ° C for 10 hours. Construction methods with high heat input, such as welding, will destroy the refinement. After welding a frame with 6000 aluminium, the heat treatment is completely redone!
That takes time and money.
Small builders usually opt for a tube from the 7000 series. No solution treatment needed, only artificially aging: four days after welding, we heat for 6 hours at 90 ° C and 4 hours at 150 ° C.
The most common quality designation for aluminium is the registration of the Aluminum Association (American). As an example we take AA 2024-T4. The main alloying element here is copper (characteristic of the 2000 series). A well-known trade name for this is duralumin.
The T4 in the "AA formula" is a thermal solution treatment up to ± 530 ° C . After this, it is rapidly cooled to 220 ° C. Then it is allowed to age naturally at 20 ° C.
The T5 treatment, has no solution step, but uses only artificially aging, i.e. heated at 160 to 190 ° C for 4 to 10 hours.
Treatment T6 gets a solution treatment and is artificially aged after that step. The yield point and the tensile strength are higher; but yield before braking decreases. To make aluminium strong, the metallurgists try all their tricks. The yield point of steel, however, is 2 to 3 times as high.
The 5000 series mainly contain magnesium. The alloy AA 5086-H18 (including Vitus Duralinox) cannot be made stronger by heat treatment. The high tensile strengths of this alloy are due to cold deformation (mixed crystal hardening, the H in the additive). The alloy can be welded, but recrystallization takes place and the strength gained is partly destroyed. Vitus glues and there is no problem.
The 6000 series contain magnesium and silicon; the 7000 mainly zinc, but sometimes also zirconium and / or scandium; the 8000 contain lithium (e.g. Reynolds X-100).
Aluminum can be welded via the TIG and MIG process with argon as shielding gas. Welding aluminum requires a lot of skill from the welder! The correct choice of welding wire is very important. The tube manufacturer will specify a certain type of welding wire for each type. The welding of heat-treated tube is generally difficult. The alloys AA 6061-T6 (magnesium-silicon) and AA 7020-T6 (zinc-magnesium) were easy to process in the first generation of tubes; the crystal structure deteriorated after welding, but was restored by aging at room temperature (this took about a month). Modern tubes are thinner and stronger and do need heat treatment.
As an example of the old series I take the Altec-ATB tube set from Columbus from 1997. This was an AA 5086 tube with a weight of 1750 grams per set. The successor Altec2 from 2005 is of the 7000 series and weighs only 1136 g (one third less!). Unfortunately, this also makes processing more difficult. No heat treatment was required for the first Altec. Altec2 requires artificial aging. Four days after welding, we heat for 6 hours at 90 ° C and 4 hours at 150 ° C. In order to obtain a sufficiently strong frame. Altec2 opts for the horizontal down tube a wall thicknesses of 2.2 / 1.0 / 1.4 mm. The manufacturer Easton has a down tube even two tenths of a mm thinner (type Sc7000: 2.0 / 0.8 / 1.2). The safety margins are small; they prescribe that no (heavy) lighting battery may be placed in the bottle cage to prevent the bottle caps from tearing out! Again, artificially aging must be done after welding.
Even more difficult to process are the AA 6061 tubes from Easton. Here, a solution treatment must be carried out at first: half an hour at 530 ° C, then cooling with hot water and artificially aging (10 hours at 180 ° C). All these post building processes cost a lot of time, energy and money. The investments involved make the production of frames with these tubes by small builders too expensive.
Gluing frames has gone out of fashion again. When we glue, we can choose a strong non-weldable tube e.g. AA 7075-T6, but keep in mind that low stiffness is the limiting factor, not tensile strength! High-quality aluminum alloys are not easily available; don't buy an unknown alloy! Many manufacturers order their tube to their own specifications directly from the aluminium factory. Aluminium available in the Netherlands is often not classified according to the American AA standard, but according to a DIN or ISO standard. In ISO, T4 is TB, T5 TE and T6 TF. Comparable are: AA 6061: DIN AlMg Si 1: ISO Al-Si1Mg; AA 7020: DIN AlZn 4.5 Mg 1: ISO AL-Zn4Mg; AA 7075: DIN AlZnMgCu 1.5: no ISO standard .
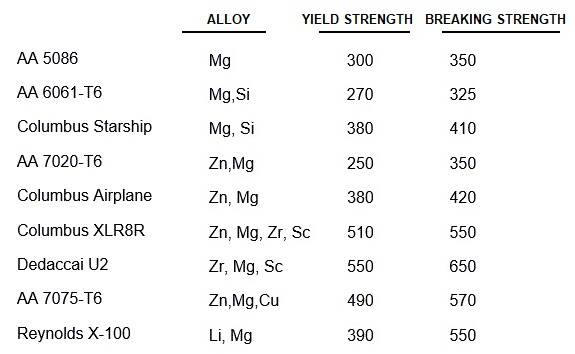
The only good welding method for high-quality aluminum is TIG welding; unfortunately, the equipment required is rather expensive. TIG welding uses a welding torch with an arc that is drawn between a tungsten tip and the workpiece, under a shielding gas like argon or helium. Like oxy-fuel welding, extra material is added with a welding wire. The view on this welding process is very good. The temperature of the arc is high, but the amount of heat input is low and the weld requires hardly any post-processing. Often it is necessary to use inert gas (backing gas) on the inside of the tube during welding. As with any welding method, the quality of the weld depends on the craftsmanship of the builder.
Some guideline values for this process. (Aluminium welding is done with alternating current!): Wall thickness 1.5 mm, electrode 1.6 mm, welding current 115 A, argon 6 l / min, welding wire 1.6 mm For AA 7000 choose a welding wire type 5356, 5180 or 5183. For AA 6000 we need welding wire 4043 or 4145. Special alloys with Scandium or Zirconium require a special welding wire supplied by the tube manufacturer!
Welding aluminium is quite laborious. We start with degreasing, for example with acetone; then the oxide layer of the tube must be sanded off with Scotch Brite or stainless steel scouring pads (no sandpaper). Then degrease again. After the last degreasing, the tube must be processed within one hour to prevent the new formation of oxides. The pre-opening between the tubes when welding should be approximately 0.5mm; more than 0.75mm can cause problems. The seam must be done in one go; start and end of the weld must be on the side (neutral line). Don't run the welding torch over the weld a second time to make corrections or to get a smoother surface. Immediately after welding, while the frame is still warm, any straightening should be done. The tube manufacturers are against filing to smooth the weld, because the risk of damage is high and the weld will not become stronger; sanding is recommended with 400 or 600 paper.
information on the Internet:
A neutral site over aluminium: http://www.bikepro.com/products/metals/alum.html (also some titanium and beryllium)
Reynolds makes aluminium tubes too: http://www.reynoldstechnology.biz/